2- Introduction
The world's population is increasing rapidly, while providing enough food for this growing population has become a significant challenge. Food is usually categorized into different classes containing fruits, vegetables, grains, proteins, and dairy [1]. Meat and other alternative forms of proteins are among the top essential foods for the human body [2]. Due to high protein content, nuts are considered one of the main substitutes for meat and a popular element of American families' food basket. From 2000 to 2016, nut consumption has increased from 2.61 to 4.8 lb per capita [3]. Based on the California Department of Food and Agriculture, per capita consumption of fruits and tree nuts is the third-largest among the major food groups, after dairy products and vegetables [4]. The United States is among the top nuts producers globally, and the State of California (CA) produces about 100% of the United States’ almonds, walnuts, and pistachios by estimated bearing acres of more than 1.53 million, 440,000, and 289,000, respectively. These orchards produced nuts with the total value of about $9 billion in 2019 [5] by accounting 10% estimated increase of the acreage since 2018. Figure 1 shows the total reported acreage of almond, walnut, and pistachio, separately, in California from 1995 to 2019. The growing trend of nut orchards is noticeable, especially for almond that has grown more than 300% during the past 25 years, with an estimated annual increase of 2-3.5% in the 2020s [6], [7]. The nut industry continues to grow, and as a result, more lands are getting under cultivation, raising demand for inputs, causing an imbalance in available and needed resources [8].
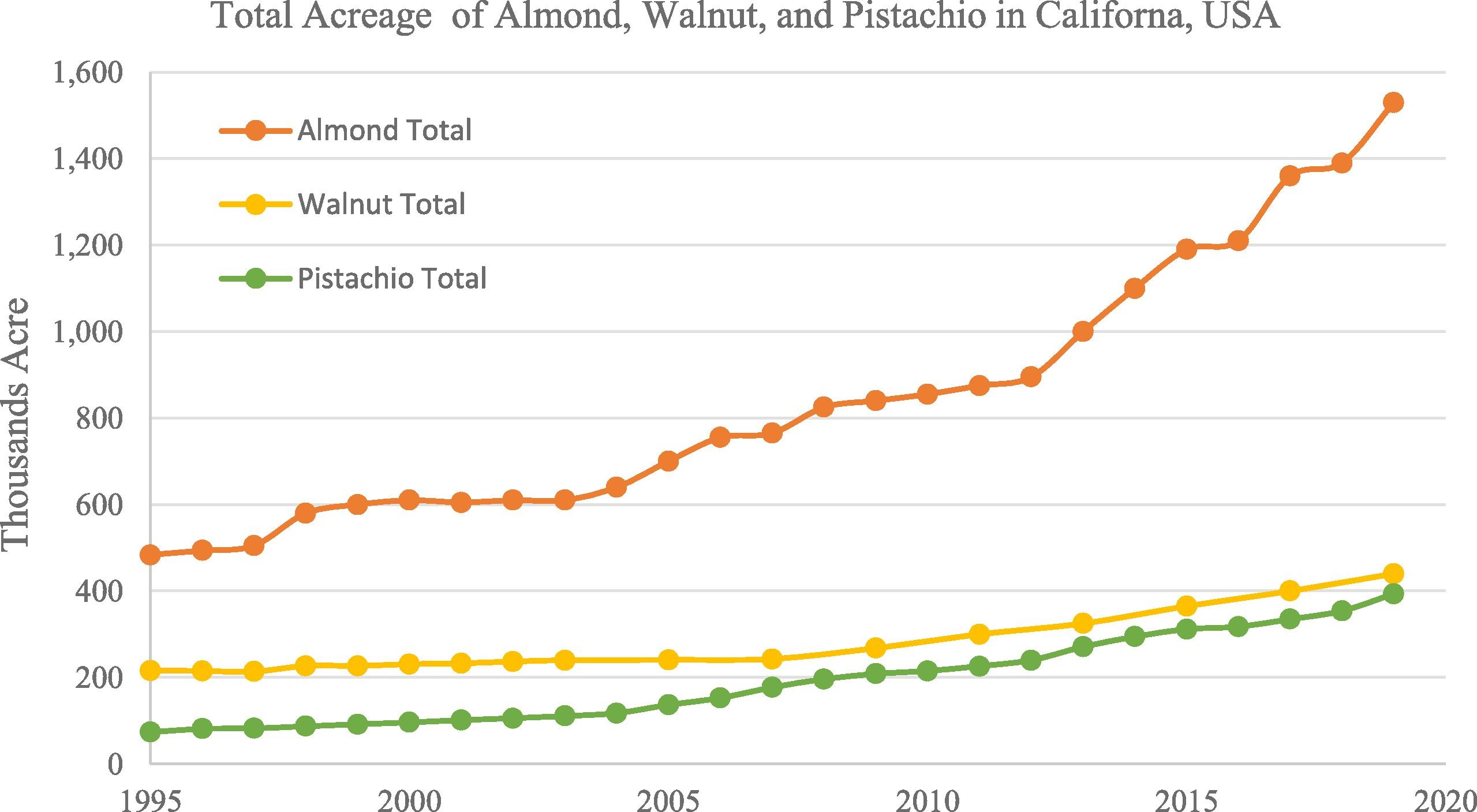
Figure 1- Increasing acreage of nuts (Almond, Walnut, Pistachio) in California, USA from 1990-2019 [7].
Limited natural resources restrict the expansion of agricultural lands and planting new orchards; therefore, optimizing production practices becomes inevitable. As a result, many researchers worldwide are looking for sustainable solutions for food production systems compatible with depleting natural resources (water, arable land) [9], increasingly extreme climate [10], stricter environmental legislation, and the emergence of new and more resistant pests and diseases [11]. For this purpose, data-driven decision-making must substitute conventional orchard management techniques to address the inefficiency of agricultural operations such as irrigation, nutrient management, pest and disease management, pruning, and harvesting.
Precision Agriculture ( PA- defined as the site-specific and timely management of crops [12]) is based on data-driven procedures to enhance agricultural efficiency by minimizing inputs and waste and maximizing yield quantity/quality while protecting the environment and ensuring sustainability. Models predicted that PA practices promise to increase the global yield by up to 67% by 2050 [13]. One crucial step of PA is data collection and interpretation that enables growers to conduct large-scale crop monitoring and determine within-field spatial variabilities; the results will be prescription maps used for variable rate application/management [4]. Large-scale data collection is mostly conducted by remote sensing techniques, the cornerstone of the entire PA process. According to ASPRS (American Society for Photogrammetry and Remote Sensing), remote sensing is defined as “the measurement or acquisition of information of some property of an object or phenomena, by a recording device that is not in physical or intimate contact with the object or phenomenon under study, e.g., the utilization at a distance”[14]. Remote sensing in agriculture refers to a non-destructive method of recording and analyzing plants' physical and physiological characteristics [15]. Remote sensing applications, in general, employ electromagnetic radiation to collect field data that can be linked to various biotic and abiotic stresses or other quantities [15].
In this manuscript, we conducted a comprehensive literature review on remote sensing and aerial imaging applications with a focus on nut production. However, most of the materials presented in this paper is applicable to other specialty crops and to a lesser extent to field crops. This paper is organized as follows: In section 3, several aerial data collection platforms commonly used for remote sensing are introduced and compared. Section 4 explores the advantages and disadvantages of different types of sensors used in remote sensing. In section 5, various applications of remote sensing data are discussed. Section 6 focuses on data processing and analysis steps, and finally, in section 7, conclusions are presented.
References:
[1] M. Campbell, “5 Main Food Groups,” Dec. 2018. https://healthyeating.sfgate.com/5-main-food-groups-3976.html (accessed Dec. 30, 2022).
[2] D. Alasfoor, H. Rajab, and B. Al-Rassasi, “Food based dietary guidelines, technical background and description,” Muscat: Ministry of Health, 2007.
[3] M. Shahbandeh, “Per capita consumption of tree nuts in the U.S. 2017,” Statista, Jan. 29, 2019. https://www.statista.com/statistics/184216/per-capita-consumption-of-tree-nuts-in-the-us-since-2000/ (accessed Dec. 30, 2019).
[4] (California Department of Food and Agriculture, “California Agricultural Statistics Review,” (California Department of Food and Agriculture, 2018. Accessed: Dec. 18, 2019. [Online]. Available: https://www.cdfa.ca.gov/Statistics/PDFs/2017-18AgReport.pdf
[5] United States Department of Agriculture, “USDA - National Agricultural Statistics Service - California - Specialty and Other Releases,” 2020. https://www.nass.usda.gov/Statistics_by_State/California/Publications/Specialty_and_Other_Releases/ (accessed Feb. 19, 2021).
[6] T. Zhao, B. Stark, Y. Q. Chen, A. L. Ray, and D. Doll, “Challenges in Water Stress Quantification Using Small Unmanned Aerial System (sUAS): Lessons from a Growing Season of Almond,” Journal of Intelligent and Robotic Systems: Theory and Applications, vol. 88, no. 2–4, pp. 721–735, Dec. 2017, doi: 10.1007/s10846-017-0513-x.
[7] (California Department of Food and Agriculture, “2018 California Almond Acreage Report,” (California Department of Food and Agriculture, Apr. 2019. [Online]. Available: https://www.nass.usda.gov/Statistics_by_State/California/Publications/Specialty_and_Other_Releases/Almond/Acreage/201904almac.pdf
[8] I. F. García-Tejero, V. H. Durán-Zuazo, and J. L. Muriel-Fernández, “Towards sustainable irrigated Mediterranean agriculture: implications for water conservation in semi-arid environments,” Water International, vol. 39, no. 5, pp. 635–648, 2014, doi: 10.1080/02508060.2014.931753.
[9] J. M. Costa, O. M. Grant, and M. M. Chaves, “Thermography to explore plant–environment interactions,” Journal of Experimental Botany, vol. 64, no. 13, pp. 3937–3949, Apr. 2013, doi: 10.1093/jxb/ert029.
[10] N. V. Fedoroff et al., “Radically rethinking agriculture for the 21st century,” science, vol. 327, no. 5967, pp. 833–834, 2010.
[11] D. A. Lubin and D. C. Esty, “The sustainability imperative,” Harvard business review, vol. 88, no. 5, pp. 42–50, 2010.
[12] B. M. Whelan and A. B. McBratney, “The ‘null hypothesis’ of precision agriculture management,” Precision Agriculture, vol. 2, no. 3, pp. 265–279, 2000.
[13] M. W. Rosegrant et al., Food security in a world of natural resource scarcity: The role of agricultural technologies. Intl Food Policy Res Inst, 2014.
[14] J. Estes, K. Kline, and E. Collins, “Remote Sensing,” in International Encyclopedia of the Social & Behavioral Sciences, N. J. Smelser and P. B. Baltes, Eds. Oxford: Pergamon, 2001, pp. 13144–13150. doi: 10.1016/B0-08-043076-7/02526-2.
[15] H. G. Jones and R. A. Vaughan, Remote sensing of vegetation: principles, techniques, and applications. Oxford university press, 2010.